What is Nuclear Fusion?
Nuclear fusion is a process in which two or more light atomic nuclei combine to form a heavier nucleus, releasing a tremendous amount of energy in the process.
This is the same process that powers the sun and other stars, where hydrogen nuclei fuse together to form helium and release an enormous amount of energy in the form of light and heat.
The Process
Setting the Scene
Picture a scorching-hot, super-dense core at the sun’s centre. Here, hydrogen atoms are under immense pressure and temperature – hotter than you could ever imagine. This extreme environment forces hydrogen nuclei to get wild and crash into each other at extreme speeds.
The Resistance
Now, hydrogen nuclei contain one proton and think of these protons as tiny, positively charged magnets. You know how magnets repel each other when you try to push them together? That’s what’s happening here. The protons repel each other due to their positive charges. But the sun is a relentless force of nature, and it doesn’t give up easily.
The Collision
To overcome the proton between repulsion, they need to be racing at such astonishing speeds that they practically collide with the energy of a thousand race cars combined! And when they finally crash, something magical occurs. Two nuclei fuse together, and voila! They create a helium nucleus. This combining of the two nuclei is called Fusion.
What can undergo Fusion?
Nuclear fusion can occur with various elements, but the specific elements that readily undergo fusion at the temperatures and pressures achievable on Earth are limited. The most common elements used for nuclear fusion are isotopes of hydrogen, such as deuterium and tritium, meaning that they have the same number of protons in their nuclei but different number of neutrons where deuterium has 2 neutrons and tritium has 3. Both deuterium and tritium are naturally occurring isotope of hydrogen however, tritium is very rare but can be produced artificially. When these two isotopes are heated to extremely high temperatures and put under intense pressure, they can fuse to form helium and release a significant amount of energy.
Deuterium-tritium (D-T) fusion is the most widely studied and the easiest to achieve with current technology. However, there are some challenges associated with tritium production and handling, as tritium is radioactive.
In addition to D-T fusion, other isotopic combinations can also undergo fusion, such as:
- Deuterium-Deuterium (D-D) fusion
- Deuterium-Helium-3 (D-3He) fusion
These reactions require even higher temperatures and pressures than D-T fusion and are more technically challenging to achieve.
An Example of the Amount of Energy Released
If 1 atom of deuterium and nucleus of tritium combined through nuclear fusion it will produce nucleus of helium, a neutron and a lot of energy:

Deuterium
Tritium
Helium
Neutron
Using Albert Einstein’s equation E=mc², we can determine that 337.6GJ of energy is released per kg of D-T fuel which consists of deuterium and tritium.
This equates to roughly the same amount of energy released from burning 14,070kg of coal and is enough energy to boil roughly 1,008,122 kettles containing 1 Litre of water!
Energy is released by nuclear fusion, when mass is converted into energy.
To use Albert Einstein’s equation E=mc², we need to calculate the amount of mass that has been converted into energy by finding the difference in masses before and after the reaction.
To do that we use a unit called the Atomic Mass Units (AMU), where 1 AMU has a mass of 1.66×10-27kg. The elements within this reaction have the following mass in AMUs:
Atom | Atomic Mass (AMU) |
---|---|
Deuterium | 2.014102 |
Tritium | 3.016049 |
Helium | 4.002603 |
Neutron | 1.008665 |
Next we need to work out the mass before the reaction takes place:

Before the reaction there is one atom of tritium and one of deuterium meaning that the total mass is 5.030151 AMU.
[2.014102 + 3.016049 = 5.030151 AMU]
We also need to find the mass after the reaction to be able to determine the difference:
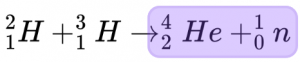
After the reaction there is one atom of helium, one neutron resulting in a mass of 5.011268 AMU.
[4.0026 + 1.008665 = 5.011268 AMU]
This means that 0.18883 AMU of mass has been converted into energy as the first law of thermodynamics that energy can not be created or destroyed it can only be transferred into other forms.
[5.011268 – 5.030151 = 0.18883 AMU]
Using Einstein’s equation the total energy release can be calculated:

This tells us that for every atom of deuterium that undergoes fusion with tritium produces 2.82 x 10-12J of energy.
We can calculate how much energy is released per 1 g of deuterium-tritium fuel by multiplying the amount of energy per atom by the number of atoms. Where first we need to determine the amount of each element we are using based on their mass proportions as an assumption to have the maximum amount of fusion reactions.
For example the amount of deuterium we will consume will be the 1 gram multiplied by the atomic mass of deuterium over the combined masses of tritium and deuterium which is 5.030151 AMU.
[2.014102 + 3.016049 = 5.030151 AMU]
This means that we will consume 0.4 g of deuterium:
And 0.6 g of tritium:
Next we need to calculate how many moles of each element are being consumed where 1 mole of deuterium will have a mass of 2.014 g and therefore we are using 0.1988 moles.
[0.4/2.014 = 0.1988 moles]
This should match the number of moles of tritium we are consuming. Tritium has a mass of 3.016 grams per mole, meaning we would be consuming 0.1988 moles.
[0.6/3.016 = 0.1988 moles]
Yes! That is the same!
Avogadro constant is the number of atoms in 1 mole which equals 6.022×1023 Atoms per Mole, meaning we have a total of 1.1972×1023 atoms.

Therefore the total amount of energy released from 1 gram of standard D-T fuel undergoing fusion is:

Applications of Nuclear Fusion
Nuclear fusion has several potential applications and it holds great promise in various fields. Some of the most notable uses for fusion include:
- Electricity Generation: The primary application of nuclear fusion is electricity generation. Fusion reactors could provide a consistent and reliable source of electricity, powering homes, industries, and communities.
- Hydrogen Production: Fusion reactors can be used to produce hydrogen, which is an essential fuel for various applications, including fuel cell vehicles and industrial processes. This can contribute to a clean and sustainable hydrogen economy.
- Medical Isotope Production: Fusion reactors can produce high-energy neutrons, which can be used to generate medical isotopes for various diagnostic and therapeutic applications in the field of medicine.
Explore Further
Choose from the articles below to continue learning about nuclear.
Radiation Dose – Measuring what can’t be seen
Radiation – A terrifying invisible killer?
The Atom – Building block of the universe
Did you know? Explore Nuclear also offers great careers information and learning resources.
Below you can find references to the information and images used on this page.
Content References
- World Nuclear Association – Nuclear Fusion Power
- What is nuclear fusion | IAEA
- Nuclear fusion: what it is, definition and example
- What Is Nuclear Fusion? Definition and Examples
- Angelo – Atomic Masses
- Nuclear fusion – Wikipedia
- Nuclear fusion – Nuclear fission and fusion – AQA – GCSE Physics (Single Science) Revision – AQA – BBC Bitesize