History of Molten Salt Reactor Development
Molten salt reactors (MSRs) have a rich and intriguing history that spans over seven decades. From their inception during the Cold War to their resurgence in the 21st century, MSRs have evolved significantly, promising a safer and more efficient future for nuclear energy.
Early Beginnings: The 1940s and 1950s
The concept of MSRs was born out of the U.S. Aircraft Nuclear Propulsion Program in the late 1940s. Researchers were exploring the potential of nuclear-powered aircraft, and the idea of using a liquid fuel reactor emerged as a promising solution. The flexibility and efficiency of molten salt as a coolant and fuel carrier made it an attractive option for this ambitious project.
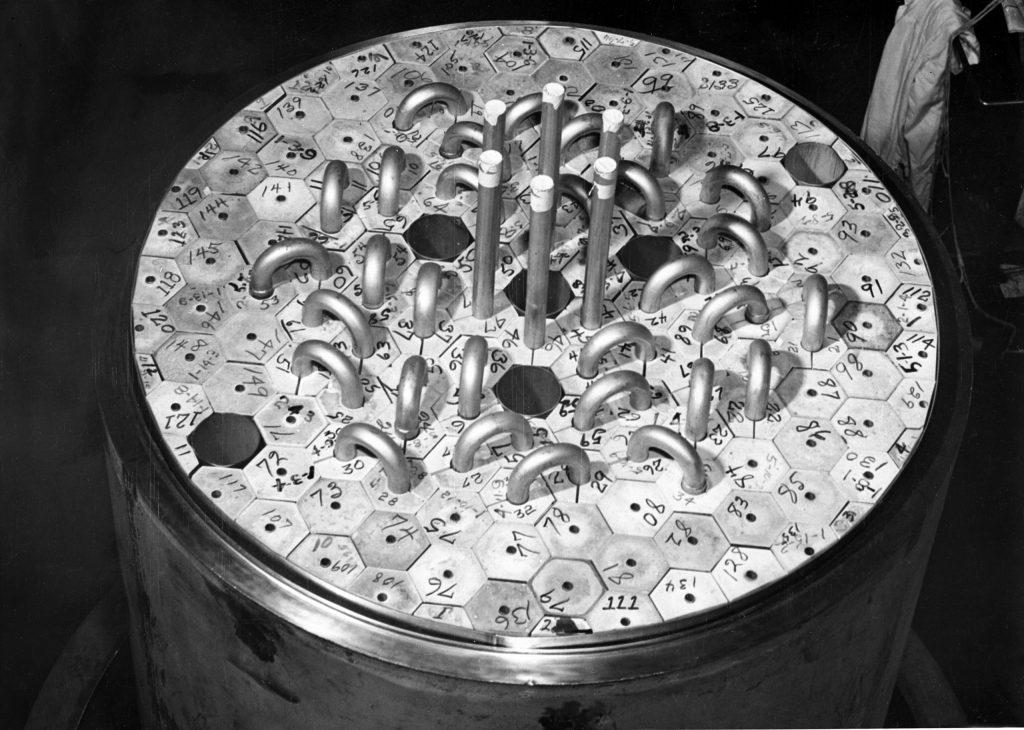
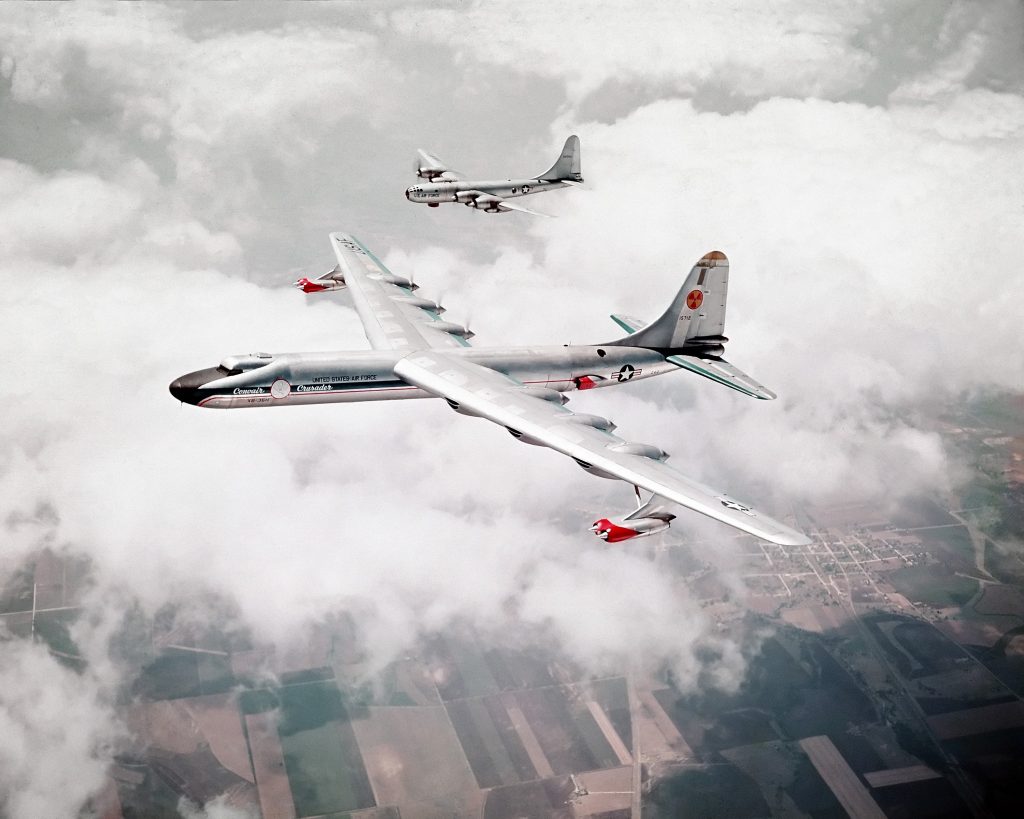
The Molten Salt Reactor Experiment (MSRE): 1960s
The most significant early development in MSR technology was the Molten Salt Reactor Experiment (MSRE) at Oak Ridge National Laboratory (ORNL) in Tennessee, USA. Construction of the MSRE began in 1962, and it achieved its first criticality in 1965. This experiment was a pivotal moment in the history of MSRs, demonstrating the feasibility of using molten salt as both a coolant and a fuel carrier.
The MSRE operated successfully from 1965 to 1969, achieving sustained operation at full power by December 1966. It was designed for a thermal output of 15 MW but operated at 7.4 MW due to imprecise nuclear cross-section data. The experiment provided valuable insights into the behavior of molten salt reactors and laid the groundwork for future developments.
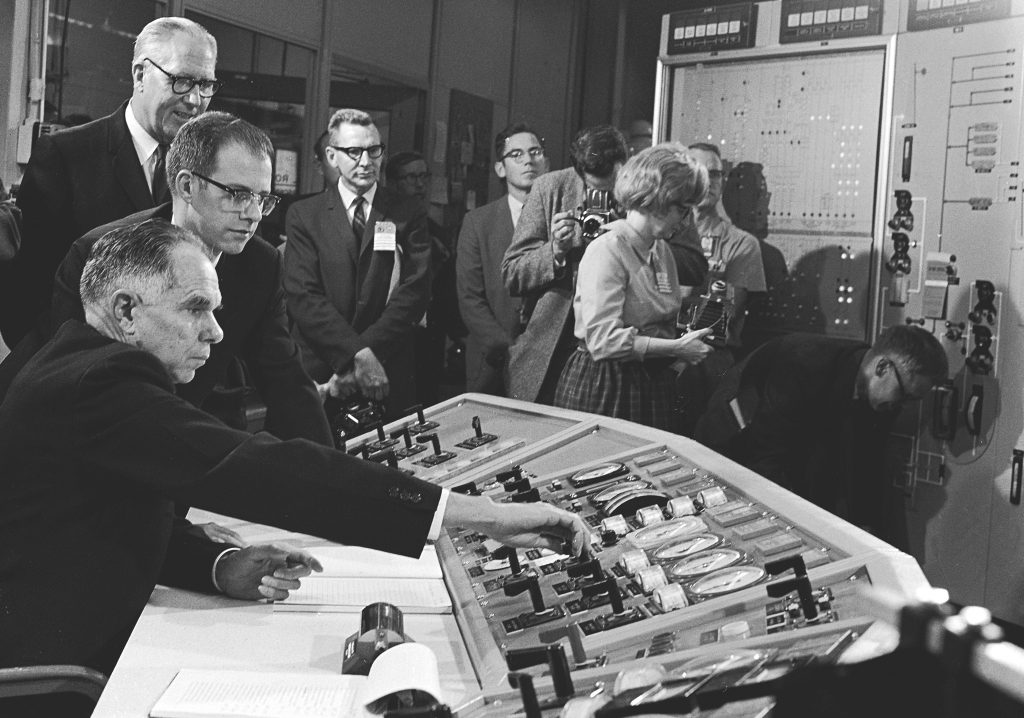
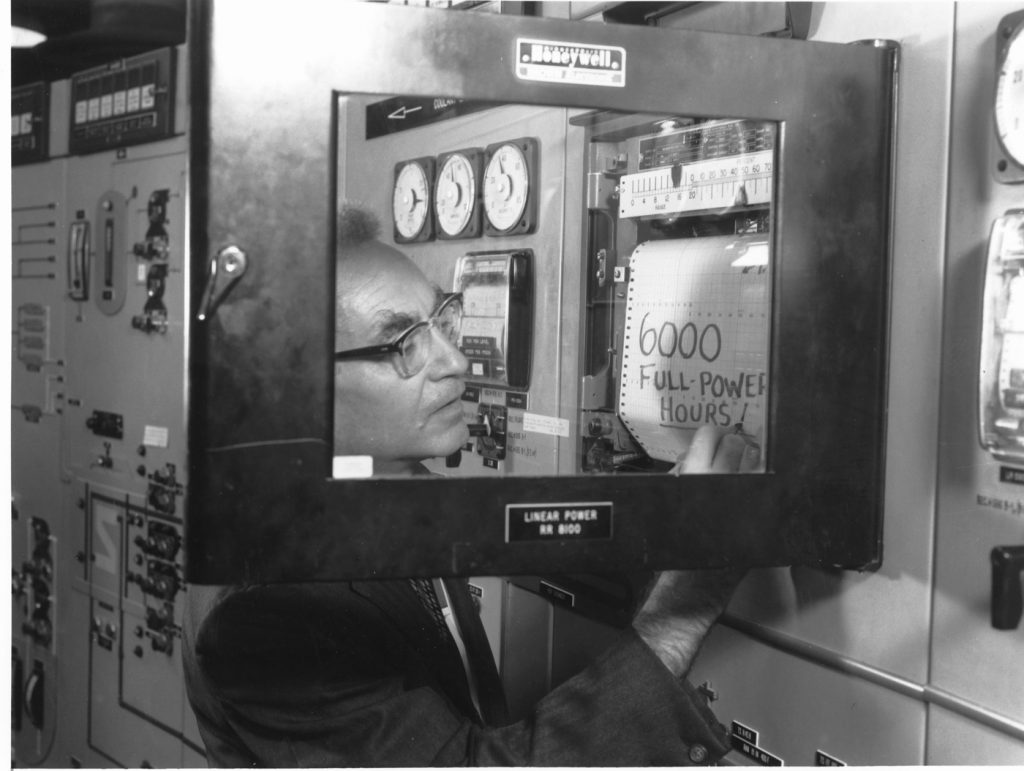
Decline and Dormancy: 1970s to 2000s
Despite the success of the MSRE, interest in MSRs waned in the 1970s as the focus shifted to other reactor technologies, such as light-water reactors (i.e. PWRs and BWRs). Research continued at a lower intensity, primarily in the United States, France, and Japan. During this period, the potential advantages of MSRs, such as inherent safety features and high efficiency, were recognised but not fully pursued.
Resurgence and Modern Developments: 2010s to Present
The 21st century has seen a resurgence of interest in MSRs, driven by the need for safer and more sustainable nuclear energy solutions. Modern MSR designs often focus on using thorium as a fuel, which is more abundant and produces less long-lived radioactive waste compared to uranium. Countries like China, the United States, and Canada are actively researching and developing MSR technology.
The International Atomic Energy Agency (IAEA) has also highlighted the potential of MSRs in achieving net-zero carbon emissions. Reports and studies from the IAEA and other organizations have underscored the benefits of MSRs, including their inherent safety features, high thermal efficiency, and the ability to utilise thorium.
How Molten Salt Reactors work
The Basics of Molten Salt Reactors
In a typical nuclear reactor, solid fuel rods are used, and water acts as the coolant. In contrast, MSRs use a liquid mixture of salts, which can include fluorides or chlorides, that are kept in a molten state at high temperatures. The nuclear fuel, such as uranium or thorium, is dissolved directly into this molten salt.
Key Components and Operation
Reactor Core: The core of an MSR contains the molten salt mixture with dissolved nuclear fuel. When the reactor is operational, nuclear fission occurs within this mixture, releasing energy in the form of heat.
Heat Exchanger: The heat generated in the reactor core is transferred to a secondary loop via a heat exchanger. This secondary loop also contains molten salt, which absorbs the heat without becoming radioactive.
Power Generation: The heat from the secondary loop is then used to produce steam, which drives turbines connected to generators, producing electricity.
Cooling System: The molten salt in the secondary loop is cooled and recirculated back to the heat exchanger, maintaining a continuous cycle of heat transfer and power generation.
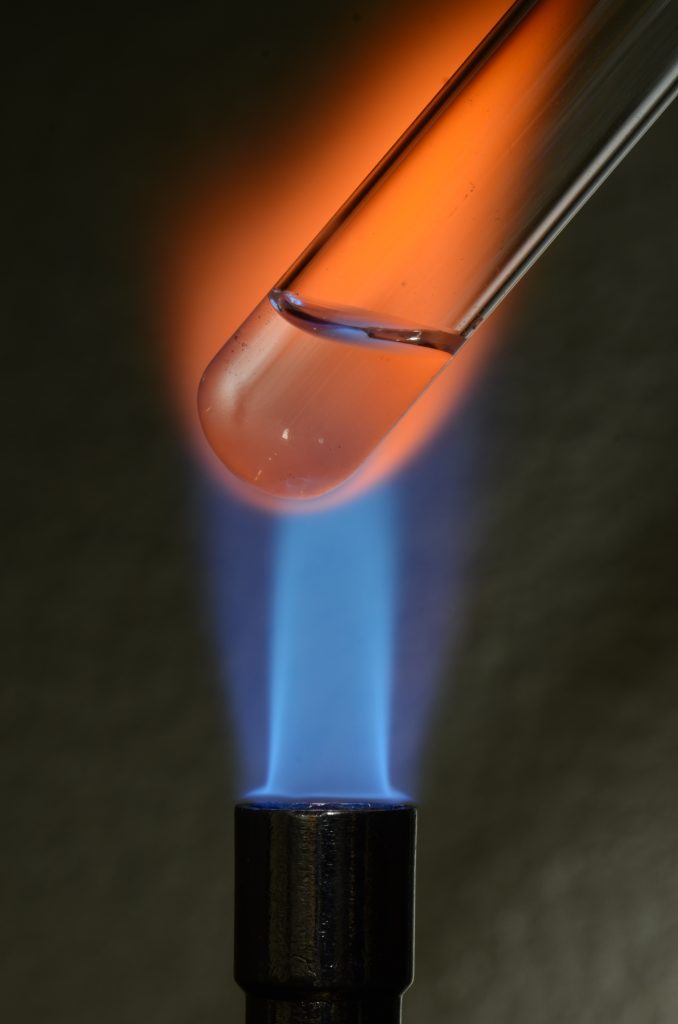
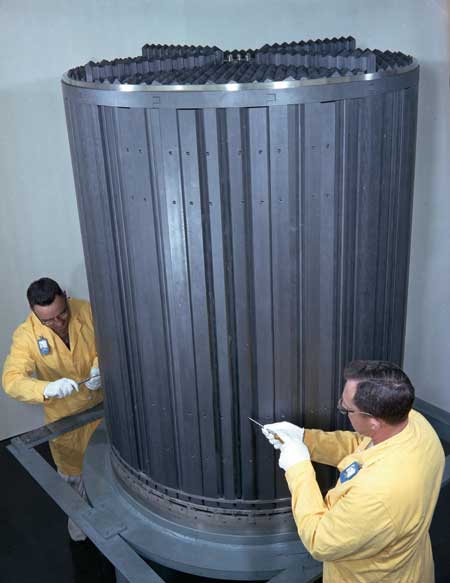
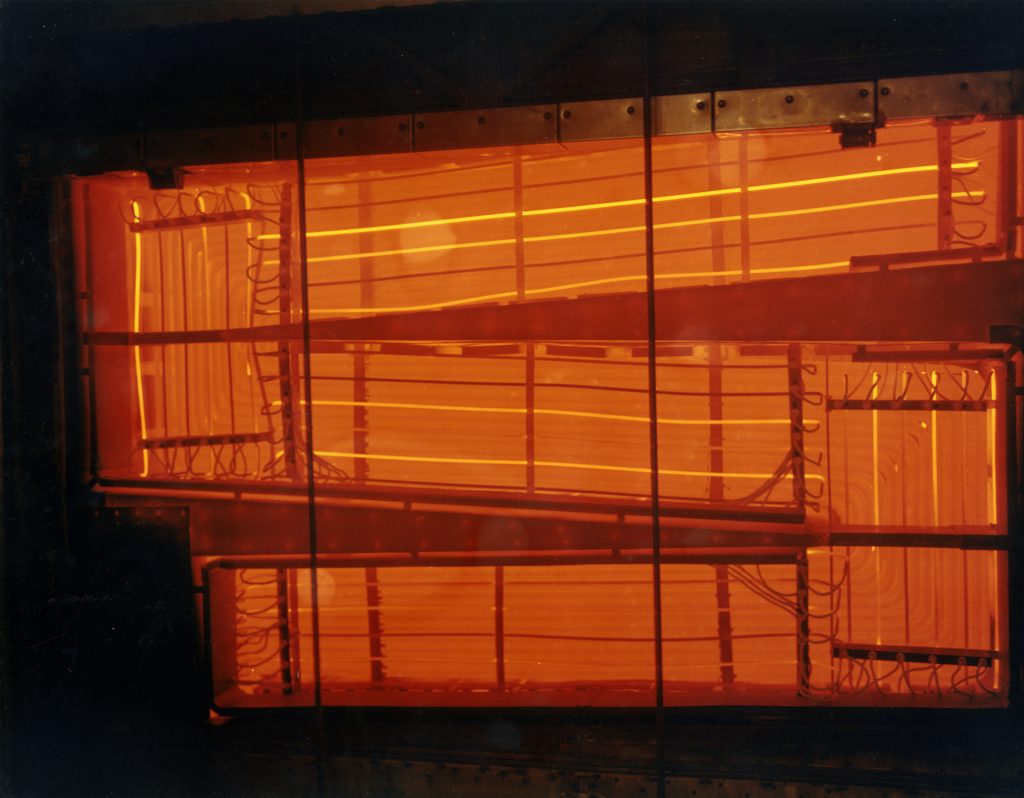
Detailed Operation and Design
Fuel Cycle: MSRs can operate on a closed fuel cycle, where the fuel is continuously reprocessed and reused. This reduces the need for fresh fuel and minimises waste. The thorium fuel cycle is particularly promising, as thorium is more abundant than uranium and generates less long-lived radioactive waste.
Online Refueling: One of the unique features of MSRs is their ability to be refueled while operating. This means that the reactor does not need to be shut down for refueling, increasing its availability and efficiency.
Corrosion Management: One of the challenges with MSRs is managing the corrosive nature of molten salts. Researchers are developing advanced materials and coatings to withstand the harsh conditions inside the reactor. For example, certain metal alloys have been found to resist corrosion better when exposed to radiation.
Emergency Drain System: MSRs are designed with a passive safety feature known as the freeze plug. In the event of an emergency, the freeze plug melts, allowing the molten salt to drain into a containment vessel where it solidifies, safely stopping the reaction.
Pros & Cons of Molten Salt Reactors
Advantages of molten salt reactors
Safety: MSRs operate at atmospheric pressure, reducing the risk of explosive pressure buildup. Additionally, the molten salt can act as a natural coolant, even in the event of a power failure, preventing overheating and potential meltdowns.
Efficiency: The high operating temperatures of MSRs, typically around 700°C, allow for more efficient electricity generation compared to traditional reactors. This means more energy can be extracted from the same amount of nuclear fuel.
Waste Reduction: MSRs can use thorium, which is more abundant and produces less long-lived radioactive waste compared to uranium. Additionally, MSRs can potentially recycle existing nuclear waste, reducing the overall amount of radioactive material that needs to be managed.
Proliferation Resistance: The design of MSRs makes it difficult to extract materials that could be used for nuclear weapons, enhancing their security profile.
Challenges and limitations of molten salt reactors
Material Corrosion: The corrosive nature of molten salts poses a significant challenge. Developing materials that can withstand these harsh conditions is crucial for the long-term viability of MSRs.
Regulatory Framework: Existing nuclear regulations are primarily designed for traditional reactors. Developing new regulatory frameworks that address the unique aspects of MSRs is necessary for their widespread adoption.
Technological Maturity: While MSRs have been studied since the 1950s, they are not yet commercially available. Continued research and development are needed to bring this technology to market.
Applications and Future Prospects
Potential applications of molten salt reactors
Electricity Generation: MSRs can be used to generate electricity more efficiently than traditional reactors, making them suitable for both large-scale power plants and small modular reactors.
Industrial Heat: The high operating temperatures of MSRs make them ideal for providing industrial heat for processes such as hydrogen production, desalination, and chemical manufacturing.
Space Exploration: MSRs’ compact size and high efficiency make them a potential power source for space missions, providing reliable energy for long-duration missions.
Future prospects of molten salt reactors
Molten Salt Reactors are gaining renewed interest as a promising technology for the future of nuclear energy. With their potential for enhanced safety, efficiency, and sustainability, MSRs are being actively researched and developed by several countries and organizations worldwide.
China: China is a major player in the development of MSRs. The Shanghai Institute of Nuclear Applied Physics (SINAP) is working on a 5 MWt prototype reactor, with plans to scale up to larger reactors. China’s research focuses on both solid fuel and liquid fuel MSRs, exploring various designs to optimize performance and safety.
China’s commitment to MSR technology is part of its broader strategy to diversify its energy sources and reduce carbon emissions. The country’s ambitious plans include developing thorium-based MSRs, which promise to be more sustainable and produce less long-lived radioactive waste.
European Union: Several European countries, including France, the Netherlands, and Denmark, are engaged in collaborative MSR research projects. The European Commission supports various initiatives aimed at advancing MSR technology. These projects focus on addressing technical challenges, such as material corrosion and fuel reprocessing, to pave the way for commercial deployment.
Canada: Canada is actively involved in MSR research, particularly in the development of small modular reactors (SMRs). These reactors are designed to be more flexible and scalable than traditional nuclear power plants. A notable project in Canada recently passed a crucial pre-licensing vendor design review, marking significant progress towards the deployment of MSR technology.
United States: The U.S. has a long history with MSR technology. Today, various companies and research institutions continue to develop MSR designs, focusing on both thorium and uranium fuel cycles.
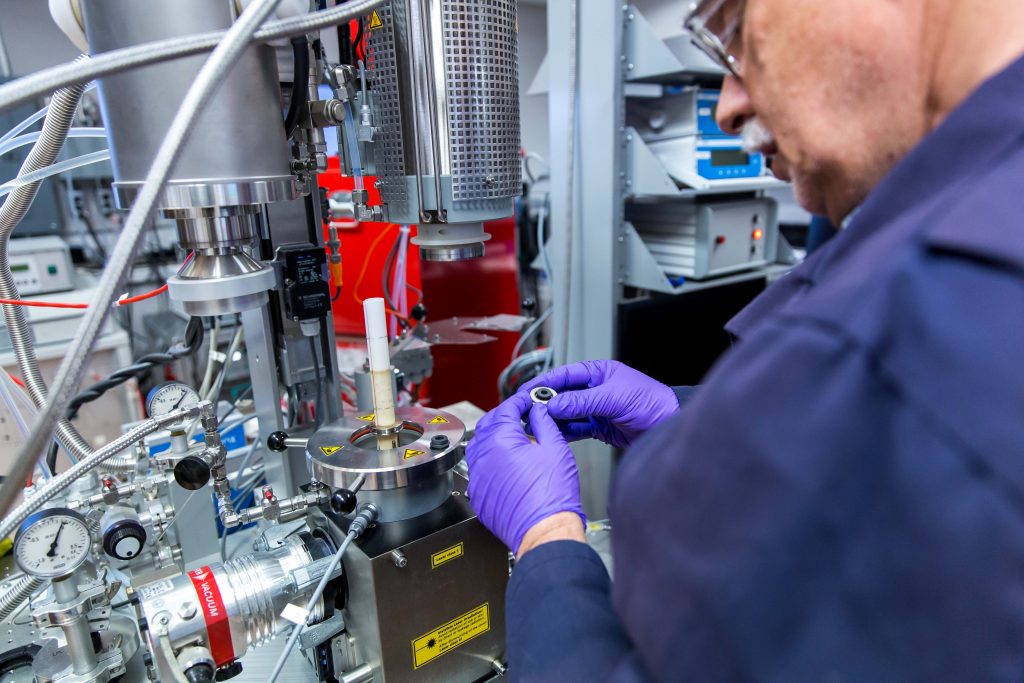
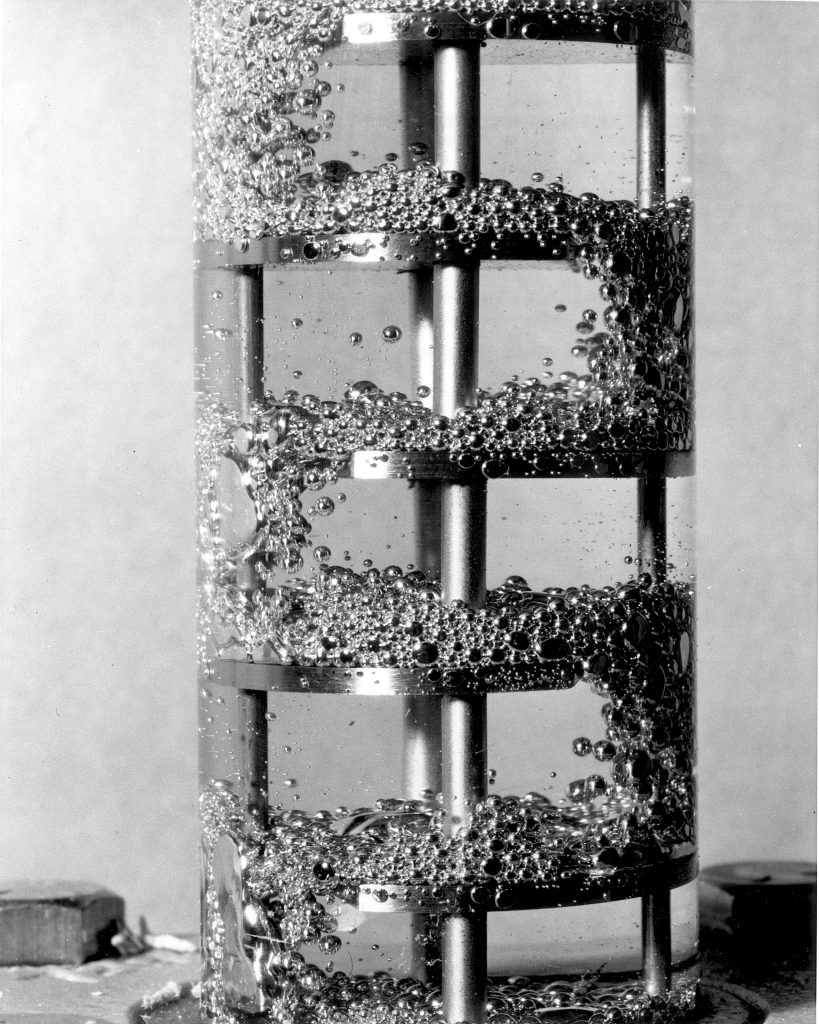
Explore Further
Choose from the articles below to continue learning about nuclear.
Advanced Modular Reactor (AMR) – The Future of Nuclear Energy Explained
Lead-cooled Fast Reactor (LFR) – from Metal to Megawatts
Supercritical Water Reactor (SCWR) – Steam, Speed and Sustainability
Gas-Cooled Fast Reactor (GFR) – High-Efficiency, Next-Gen Nuclear Power
Did you know? Explore Nuclear also offers great careers information and learning resources.
Below you can find references to the information and images used on this page.
Content References
- Molten-salt reactor – Wikipedia
- Promising designs for nuclear power reactors using molten salt | MIT Energy Initiative
- Molten salt reactor – Energy Education
- Molten salt reactors (MSR) | IAEA
- Full article: Design study of molten-salt-type reactor for powering space probes and its automated start-up (tandfonline.com)
- Molten Salt Technology Platform – National Nuclear Laboratory (nnl.co.uk)
- Molten Salt Reactor Technology Development Continues as Countries Work Towards Net Zero | IAEA
- Status of Molten Salt Reactor Technology | IAEA
- Kairos Power begins work on New Mexico salt production facility – World Nuclear News (world-nuclear-news.org)
- Generation IV Nuclear Reactors – World Nuclear Association (world-nuclear.org)
- GIF Portal – Home – MSR (gen-4.org)
Image References
- Molten Salt Test Tube – Brian Kelleher – CC BY-SA 3.0
- MSRE from above – Oak Ridge National Laboratory – Public Domain
- MSRE Core – ORNL – Public Domain
- MSRE Construction – Oak Ridge National Laboratory – CC BY 2.0
- Extraction Column – ENERGY.GOV – Public Domain
- MSRE Model – ENERGY.GOV – Public Domain
- Molten Salt Reactor Schematic – US Department of Energy Nuclear Energy Research Advisory Committee – Public Domain
- MSRE 6000 hours – (unspecified) – Public Domain
- Heat Exchanger – Oak Ridge National Laboratory – CC BY 2.0
- Aircraft Reactor Experiment – Oak Ridge National Laboratory – CC BY 2.0
- Glenn Seaborg – Oak Ridge National Laboratory – CC BY 2.0
- Advanced Reactor Research (Image 1) – Canadian Nuclear Laboratories – CC BY-ND 2.0
- Advanced Reactor Research (Image 2) – Canadian Nuclear Laboratories – CC BY-ND 2.0
- Convair NB-36H – USAF – Public Domain
- MSRE Diagram – Oak Ridge National Laboratory – Public Domain